Current Research
This is what we are working on now.
While the extraocular muscles play complex roles in ocular rotation and alignment, extraocular muscle forces must mechanically balance against the eyeball, optic nerve, and a network of suspensory tissues within the orbit. The effects of eye rotation are concentrated at the optic disc and propagate into surrounding tissues that are visually critical. The optic nerve both contributes to extraocular muscle loading, and is in turn, loaded by the extraocular muscles potentially undergoing repetitive strain injury contributing to:
- Strabismus, the misalignment of the eyes often associated with double vision
- Normal tension glaucoma
- Extreme nearsightedness, or pathologic myopia, the elongation and deformation of the eye causing nearsightedness.
The Ocular Motility Laboratory has pioneered the use of multiple techniques to study these important ocular health problems. In June 2024, the National Eye Institute renewed support of the laboratory’s research for a cumulative total of 38 years, through April 2029. This laboratory conducts three interrelated projects to clarify the ocular motility contribution to these common visual disorders.
Investigations into the causes of strabismus, which is misalignment of the eyes
Investigators use clinical alignment measurements, magnetic resonance imaging of the extraocular muscles, and computational simulations to clarify the causes of strabismus and optimize corrective strabismus surgery.
- Eye rotations. Using magnetic resonance imaging, investigators evaluate the size and position of extraocular muscles and their contractile changes during vertical and horizontal eye rotations. Magnetic resonance imaging thus indicates function of individual extraocular muscles.
- Ocular counter-rolling during head tilting. Repeating magnetic resonance imaging in people lying with right and left sides down, investigators evaluate the size of and position of every extraocular muscle during change in head tilt relative to gravity, a critical input in daily life and in diagnosis of complex vertical strabismus that causes double vision.
Characterization of in-vivo eye deformations, or strains, during eye rotation
Most current ocular imaging is done only when looking steadily straight ahead, a situation that is decidedly unnatural. In this project, investigators characterize the mechanical effects of natural horizontal and vertical eye rotations to test the hypothesis that such loading deforms the optic nerve and peripapillary retina, constituting a plausible driver of disc and globe changes in normal development, normal tension glaucoma, and severe nearsightedness.
- Static strains. During maintained eccentric fixations, investigators quantitatively image optic nerve, peripapillary retina and choroid, and vascular deformations using optical coherence tomography and confocal scanning laser ophthalmoscopy. Investigators emphasize shearing between the retina versus underlying choroid and sclera.
- Dynamic strains. Using cinematic, high-time-resolution scanning laser ophthalmoscopy, investigators test the hypothesis that local strains during flight of fast eye movements vary dynamically, substantially surpassing those during steady gaze.
Computer modeling and validation of the biomechanics of eye rotation
While concentrations of stress and strain in local regions of the eye and optic nerve are critical to ocular health, these cannot be understood without accounting for both ocular rotational properties, as well as structural and material properties of the globe, extraocular muscles, and a complex network of extraocular connective tissues in the orbit.
- Computer modeling. Extending existing simplified models, laboratory investigators use supercomputers to develop a full, three-dimensional model of the eye, extraocular muscles, and orbital tissues using the computational simulation technique known as finite element modeling, incorporating measurements of individual anatomical variations from magnetic resonance imaging, as well as explicit scleral, choroidal, and retinal layers. Investigators simulate extraocular muscle contraction using explicit algorithms appropriate to muscle and implement observed human viscoelastic and hyper-elastic tissue properties.
- Post-mortem tissue characterization. Investigators measure the whole globe and local tensile properties of fresh post-mortem, donated human eye specimens with long, attached optic nerves. First, investigators using digital image correlation, validate finite element modeling simulations of vertical duction of whole eyes with attached optic nerves subjected to bidirectional vertical rotation under varying intraocular pressure. Second, investigators consider biomechanical characterization by quantitatively characterizing the tensile creep and stress relaxation behavior of the specimen’s optic nerve, optic nerve sheath, and multiple regions of sclera immediately after digital image correlation.
- Biomechanical modeling of ocular statics. Using 3D finite element modeling, investigators model stresses and strains during a range of maintained smaller and larger horizontal and vertical eye rotations, including convergence to read and everyday glancing about. Investigators simulate medical and surgical therapeutic approaches. The first approach addresses glaucoma. This modeling indicates the extent to which the full repertoire of eye movements could generate the patterns of stresses and strains that contribute to blinding damage to the optic nerve. The second approach considers nearsightedness. The hypothesized role of optic nerve loading in myopia requires topographic match of finite element modeling predicting local stress and strain with typical patterns of myopic change in the eye.
- Biomechanical modeling of ocular dynamics. Investigators validate the dynamic finite element modeling by simulating the flight of fast horizontal and vertical eye rotations over a range of smaller and larger angles that generate the static and dynamic strains observed in the previous project where investigators characterize in-vivo eye deformations, or strains, during eye rotation. This may ultimately lead to rational disease treatments. The first approach studies glaucoma. Finite element modeling incorporating the range of anatomical and tissue properties typical of normal tension glaucoma indicate the extent to which the full repertoire of eye movements could contribute to optic nerve disease. The second approach examines nearsightedness. The hypothesized role of optic nerve loading in axial myopia requires a topographic match in representative individual cases with finite element modeling predicting local stress and strain in the eye.

Fig. 1. Nexus of eye movements and common eye diseases. Applies to all three projects.
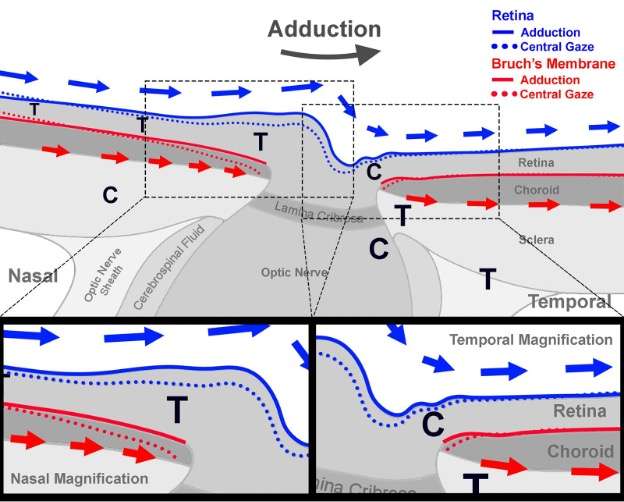
Fig. 2. Tethering by the optic nerve when looking towards the nose causes shear and strain in the retina and optic disc. Applies to projects two and three.
Image by: Park J, Moon S, Lim S, Demer JL. Scanning laser ophthalmoscopy demonstrates disc and peripapillary strain during horizontal eye rotation in adults. Am. J. Ophthalmol. 254: 114–127, 2023.